DNA
DNA carries the information that makes life possible. It is this cycling of information that we have been focusing on during the last section on meiosis, mitosis and heredity. How is it that DNA communicates that information that it is carrying. That is the objective of this section.
DNA structure
DNA is comprised of two polynucleotides, thus DNA is described as being double a stranded molecule. The two strands of DNA are not covalently bonded however, so DNA is really two molecules, but we will always refer to it as one molecule. The DNA polynucleotide is comprised of four different nucleotides.
- What does DNA stand for? Where does it get that name?
- What are the names of the four bases of which DNA is comprised?
- Which are pyrmidine bases; which are purines?
- What kinds of bonds occur between individual nucleotides; which kind occur between the two strands of DNA.
- Which base pairs are complementary in a double stranded DNA molecule? (Two complementary bases is called a basepair.)
- DNA is a bidirectional molecule. From where does the terminology 5’ and 3’ come?
DNA replication
The complementary nature of DNA suggested to Watson and Crick a mechanism for DNA replication. DNA replication (like most processes we have learned) can be divided into multiple steps that we understand are actually occurring simultaneously.
In the first step of DNA replication, DNA strands must be unwound.
- What 3 enzymes play a role in this aspect of the process? What is the function of each of these enzymes?
In the second step of DNA replication, an RNA primer must be laid down. The enzyme, DNA primase, creates this short segment of DNA. In the third step, DNA polymerase adds nucleotides to the end of the RNA primer.
- Why must an RNA primer be laid down before DNA polymerase can begin to build the new single strand of DNA?
- What determines the order in which nucleotides are added to the new single strand of DNA?
- DNA polymerase is an enzyme. From where does this enzyme get its energy to catalyze this chemical reaction?
In the final step, when DNA polymerase meets up with another RNA fragment (where another replication event had started), it will degrade the RNA primer and replace it with DNA nucleotides. An enzyme called DNA ligase connects the two single strands of DNA that have met end to end. (See Figure 14.10.
To add one level of complication, the synthesis of DNA strands does not occur in the same direction on each strand. DNA polymerase can add nucleotides to only the 3’ end of another nucleotide, therefore DNA synthesis must take place in different direction for each strand. In this case there is a leading strand and a lagging strand. The leading strand is replicated in one smooth motion and grows toward the replication fork. The lagging strand is replicated in short bursts, in which each burst must be connected by DNA ligase. The lagging strand pieces grow away from the replication fork.
Imagine you have the following double-stranded DNA molecule.
3’CGATTAGTCTCTGGGTACAAGTTGGAGAGAAACCATACTCCGTAAATCAGAGA5’
5’GCTAATCAGAGACCCATGTTCAACCTCTCTTTGGTATGAGGCATTTAGTCTCT3’
- If the origin (start) of replication is the right end of the molecule, show with an arrow the direction of DNA replication on each strand.
- Knowing this, which strand in this example will be the leading strand, which one would be the lagging strand.
I would suggest “opening up†this DNA molecule and drawing a picture of the leading and lagging strands that might look something like Figure 14.9.
- How many new DNA molecules will be present after replication of this one DNA molecule?
- Show the products that will result from the replication of this DNA strand. Indicate which strand in each daughter molecule served as the template during replication.
- How do we know that each “daughter†molecule contains one entire strand of from the “mother†molecule?
Remember that the entire DNA molecule is not replicated from just one starting point. A single DNA molecule is 100,000’s of basepairs long. There are many starting points along the DNA molecule and eventually all of the replication bubbles are joined and a new DNA molecule is released.
It is extremely important that you understand the concept that DNA replication is possible because the DNA molecule is double stranded and because of the complementary relationship of the bases within each strand.
- During which phase of mitosis does DNA replication take place?
- During which phase of meiosis does DNA replication take place?
Transcription
The individual nucleotides of DNA are arranged in such a way that they contain the information needed to make RNA and proteins that will give rise to the physical characteristics we call phenotypes (they give rise to life). Where this information starts within the DNA molecule is often complex. The language does have a direction, but the information may be contained on the upper or the lower strand (but it does not change strands in the middle of a gene). The information may also overlap within or between strands. And all of these may apply simultaneously. Usually only one strand of in a protein coding region of DNA is used as a template (but simpler rules have been broken). The template strand of DNA is the strand of DNA that is complementary to the mRNA product. The coding strand of DNA is the strand that looks like the mRNA product except with thymine rather than uracil (but looking like the mRNA product has no known biological relevance).
The transfer of information from DNA to phenotype starts with transcription. During transcription, the RNA molecule is made.
- What does RNA stand for? Where does it get that name?
- What are the names of the four bases of which RNA is comprised?
- Which of these are purines; which are pyrimidines?
- Is RNA single stranded or double stranded?
To initiate RNA transcription, the enzyme DNA-dependent RNA polymerase recognizes a specific sequence of nucleotides that are called the promoter. This sequence of nucleotides may be 5-40 bases long. (Promoter sequences do not at all resemble the codons we will learn about later.) The second stage of transcription is elongation during which one strand of the DNA copied as an RNA molecule. When the RNA polymerase reaches another signal sequence called the terminator, then the RNA polymerase falls off of the DNA and the RNA and elongation is terminated. Eukaryotic cells continue to transcribe beyond the terminator for 30-40 nucleotides. For all practical purposes, the area bounded by and including the promoter and the end of the transcription product is our gene. In our chromosome, let’s assume our promoter is TCTCT and the terminator is AATC. Assume this is a eukaryotic cell.
- What is the direction of RNA synthesis, 5’ to 3’ or 3’ to 5’? Said another way, what is the direction in which DNA is read?
3’CGATTAGTCTCTGGGTACAAGTTGGAGAGAAACCATACTCCGTAAATCAGAGA5’
5’GCTAATCAGAGACCCATGTTCAACCTCTCTTTGGTATGAGGCATTTAGTCTCT3’
- Assuming that the upper strand of DNA is the template, there is one promoter. Transcribe this gene which will not include any of the promoter sequence, but will include the terminator sequence. Show the 5’ and 3’ ends of the RNA product. Call this gene 1.
- Assuming that the lower strand of DNA is the template, there are two promoters. Transcribe these genes which will not include any of the promoter sequence, but will include the terminator sequence. Show the 5’ and 3’ ends of the RNA product. Call these genes 2 and 3.
If you find a gene that goes from right to left, write it down from left to right. However, do not change the letters. If you choose not change the direction of your gene, always remember to read it from the correct direction!
(Note: It is not rare to find one gene embedded inside of another on the same strand. However, I am not aware of an example in which two genes are embedded on opposite strands. I did this just to conserve space—and we are just beginning to understand how the genome is organized, so it could very well exist.)
Given that RNA polymerase is an enzyme, it should make sense that more than one RNA molecule is made from each gene. 10’s to 100’s of messenger RNA molecules are made when a gene is “turned onâ€.
After transcription, the messenger RNA molecules (mRNA) are sent out of the nucleus. The information the mRNA carries is translated into a protein by the ribosomes.
Translation
Protein translation is conceptually similar to replication and transcription, but there are a few more players that make the process seem more difficult. The key words from this section are:
mRNA, codon, start, stop, ribosomes, tRNA, anti-codon, amino acid, polypeptide.
Starting with the mRNA. Within the mRNA is the information required to make a protein. Like the DNA, there is a place where translation starts (initiates) and where it stops (terminates). The start and stop sites are not necessarily at the beginning and end, respectively, of the mRNA transcript. mRNA has leader and trailing sequences that are not used by the translation machinery to directly code for protein, but probably serve important regulatory functions. In the case of translation, the start and stop sites are much easier to recognize. To recognize them, we must examine the codon.
The nucleotide is the letter, and the the codon is the word of the mRNA transcript. Each word is comprised of three letters. When there are only four letters in the alphabet, there are 64 three letter combinations. Each combination of letters stands for a different word in a protein to which it is being translated. However, there are only 20-plus words in the protein language, therefore some words in the RNA language are used more than once.
Figure 15.6 shows all of the RNA and protein words. Do not let the table format make this look any different than a translation dictionary. The words could be alphabetized just like a dictionary, but that would make for a more difficult to use key. The table is merely a convenient format for the user. Have this table handy for the following questions.
When mRNA is present, the small subunit (half) of the ribosome will attach to it. When it finds the start site of translation, the large subunit of the ribosome (other half) will also attach the mRNA. The large subunit contains a landing pad for the transfer RNA (tRNA). The tRNA is a single stranded RNA molecule (made by another gene), that has a protein-like activity (it will never be translated either). The transfer RNA carries in individual amino acids that are used to build the protein. The tRNA has within its sequence, a three nucleotide anti-codon that will complement the codon of the mRNA, so that the correct amino acid is delivered. Because there are 20-some amino acids, there must be 20-some tRNAs—but there are 64 codons. How does this work? Upon examining the codon table, you will notice that some amino acids are coded by more than 1 codon. In almost each case it is the 3rd nucleotide that differs. It turns out that the matching of the anti-codon of the tRNA to the codon of the mRNA does not have to be perfect. For example, look at proline. The two bases CC will suffice for proline. There are no other amino acids that correspond to two CC nucleotides. This is called the wobble hypothesis. The codon usage is nearly universal to life as we know it.
Translation requires the participation of hundreds of macromolecules. One of the more complex players is the ribosome. The ribosome is a structural protein. Knowing the A,P and E sites helps to understand the dynamic nature of translation. Besides being a landing pad for the tRNA molecules, the ribsome holds the mRNA and it holds ribozyme. Ribozyme is the name of the enzyme that catalyzes the formation of a peptide bond between each new amino acid and the growing polypeptide. Ribozyme is not a protein—it is an RNA molecule.
Using your transcript from gene 1, identify the first AUG which will be where translation initiates. This AUG indicates the translation start site; translates to methionine and indicates the “frame†in which the sequence is read. If a frame is not established and if every word is 3 letters long, can you see how this might impact the translation?
Now underline the next three letters after the AUG. This codon is the next word. According to your table, to what amino acid does it translate? Phenylalanine. While sitting side by side in the A site (phenylalanine) and the P site (methionine) of the ribosome, this amino acid is covalently bonded to the previous amino acid, to create a polypeptide. This is the beginning of elongation.
Termination will occur when an in-frame stop codon is found. A release factor identifies these codons and when the release factor is recognized by the A site, then the ribosome complex falls apart.
- Now continue this process and provide the translation of your RNA transcript. At some point you will come to a termination codon. Make sure it is “in frame†with your start codon. There is where the translation stops. Write the primary sequence of the protein. Hint: Start and Stop are not the names of amino acids.
Notice that you did not use all of the information in the mRNA transcript. The cell may use, it but we are not actually sure why there is extra information. The actual region of the gene that is translated is called the coding region. (new word).
- Repeat the translation process with transcripts 2 and 3. Write the primary sequence of the proteins.
Congratulations, you have made protein. How long did it take you? It takes a bacteria 18 seconds to make a protein that is 360 amino acids long. This is because the same RNA may be translated by more than one ribosome complex and done so simultaneously. See Figures 16.8, 16.9.
Mutations
Based upon the primary sequence of the protein, the protein will fold and carry out its role in the cell. Mutations are not necessarily bad, and what we may call a mutation is not necessarily the “alternate†version of the gene. Sometimes the mutated form is just not the first form of the gene discovered. In general a mutation is a difference in the DNA sequence. Alleles are versions of the same gene that differ in their DNA sequence. We would not necessarily call brown eyes a mutated form of blue eyes, would we?
There are three kinds of mutations: Silent, missense and nonsense. Mutations are usually introduced as a result of a mistake during DNA replication. Considering the rate and number of DNA molecules replicated, there are very, very, very few errors.
Here is our “chromosome†again.
3’CGATTAGTCTCTGGGTACAAGTTGGAGAGAAACCATACTCCGTAAATCAGAGA5’
5’GCTAATCAGAGACCCATGTTCAACCTCTCTTTGGTATGAGGCATTTAGTCTCT3’
- Imagine that during replication the bolded A in the upper strand was substituted with a G. How would that impact the primary sequence of the protein coded by gene 1. What kind of mutation is this? Would this result in a new “version†of gene 1? How about if it were changed to a C or a T?
So there is a little room for error…
- But imagine the underlined A in the upper strand was changed to a G. How would that impact the primary sequence of the protein coded by gene 1. What kind of mutation is this? How about if it were changed to a C or a T? What kind of mutations are these? Do they have the same or different impacts? Would this result in a new “version†of gene 1?
- Finally, what would happen if the underlined A was deleted? How would that impact the primary sequence of the protein coded by gene 1. What kind of mutation is this? Would this result in a new “version†of gene 1? What if an additional A were added in just before the underlined A? What kind of mutation is this? Would this result in a new “version†of gene 1?
For additional practice, how would these same changes impact genes 2 and 3?
For more practice make up your own genes and make your own proteins. Have someone check your answers.
Transcription and translation is the basic mechanism by which information is transmitted from DNA to the rest of the cell. However, just like any form of communication, there are many subtleties. (In human communication we can think of word choice, timing or body language). Figure 18.1 gives us a starting glance at how the information transfer from DNA to protein can be modified along the way as to alter the amount, time and location of information expression, for example. There are examples of each of these mechanisms to regulate gene expression. However, as general concepts we are just beginning to understand how truly complex and beautiful the genome really is.
Looking for a similar assignment? Our writers will offer you original work free from plagiarism. We follow the assignment instructions to the letter and always deliver on time. Be assured of a quality paper that will raise your grade. Order now and Get a 15% Discount! Use Coupon Code "Newclient"
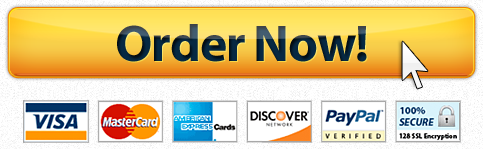